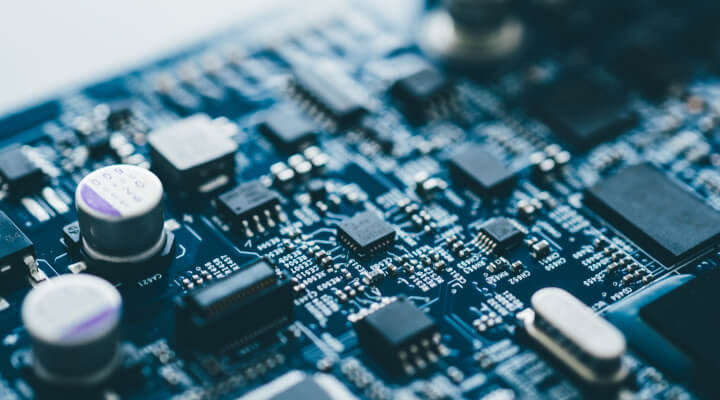
Table of Contents
- Introduction
- Key Figures in Power Electronics: Nikola Tesla and Thomas Edison
- The Dawn in the History of Power Electronics
- The Mercury Arc Rectifier Era
- Vacuum Tubes: Controlling Electricity Flow
- The Semiconductor Revolution
- Technological Challenges and Solutions in Power Electronics
- Miniaturization and Efficiency in Power Electronics
- The MOSFET Breakthrough
- The Advent of IGBTs
- Electrification and Power Electronics
- The Future: GaN and SiC Technologies
- Conclusion
Power electronics, a critical discipline within electrical engineering, orchestrates the efficient management and conversion of electrical power, serving as the backbone for a myriad of modern technological applications. This field integrates principles of electronics and power engineering to develop systems that control the flow of electrical energy, ensuring that devices from microprocessors to electric vehicles operate with optimal efficiency and reliability. For a deeper understanding of this foundational technology, consider exploring What is power electronics?, which offers a comprehensive overview.
Introduction
The history of power electronics, a critical discipline within electrical engineering, orchestrates the efficient management and conversion of electrical power, serving as the backbone for a myriad of modern technological applications. This field integrates principles of electronics and power engineering to develop systems that control the flow of electrical energy, ensuring that devices from microprocessors to electric vehicles operate with optimal efficiency and reliability.
The evolution of power electronics marks a significant trajectory from foundational concepts to complex, advanced applications. Initially focused on basic rectification and simple control mechanisms, the field has expanded to encompass sophisticated techniques in power modulation, energy storage, and high-frequency power conversion, driven by the relentless pursuit of efficiency and miniaturisation.
As we explore the nuances of power electronics, we delve into a domain where semiconductor devices, such as MOSFETs and IGBTs, play pivotal roles. These components are the building blocks that enable the precise control and conversion of power, catering to the demands of high-performance computing, renewable energy systems, and beyond. The journey from basic diodes and thyristors to today’s cutting-edge wide-bandgap semiconductors illustrates the field’s dynamic evolution, highlighting its indispensable role in pushing the envelope of technological progress.
Key Figures in Power Electronics: Nikola Tesla and Thomas Edison
Before delving into the individual contributions of the titans in the field of power electronics, it’s essential to recognise the monumental impact of Nikola Tesla and Thomas Edison. These two inventors, whose rivalry and brilliance lit the path for modern electrical engineering, laid the foundational stones of what would become a transformative era in power electronics. As we explore their legacies, we begin with Nikola Tesla, a visionary whose pioneering work on alternating current (AC) not only challenged the prevailing norms but also set the stage for the electrification of the world.
Nikola Tesla: The Pioneer of Alternating Current
In the history of power electronics, Nikola Tesla stands as a towering figure, with his birth in 1856 marking the beginning of a new era. Tesla’s contributions to power electronics are monumental, with his development of alternating current (AC) systems being his most notable achievement. This innovation provided a more efficient means of transmitting electrical power over long distances compared to the direct current (DC) systems of his time. Tesla’s work laid the foundational principles for modern power distribution, and his vision extended to wireless communication and energy transfer, concepts that are still being explored today.
Nikola Tesla was the mastermind behind the creation of the first hydroelectric power station at Niagara Falls, New York, which began generating electricity in 1895. This marked the start of the electrification process in the United States and subsequently across the globe. Presently, Tesla’s alternating current (AC) electricity is illuminating and energising the world.
Thomas Edison: The Proponent of Direct Current
Thomas Edison, an American inventor born in 1847, is often celebrated for his development of the electric light bulb. However, his influence on power electronics extends beyond this singular invention. Edison was a staunch advocate for direct current (DC) for electrical power distribution. Despite the eventual widespread adoption of AC systems, Edison’s work on DC contributed significantly to the development of power systems, especially in the context of urban electrical grids and power stations. His innovations in electrical generation and distribution systems laid the groundwork for modern electrical infrastructure.
Historical and Societal Catalysts for Innovation
The late 19th and early 20th centuries were a period of rapid industrialization and technological innovation, setting a fertile ground for advancements in power electronics. The societal need for more efficient lighting, communication, and manufacturing processes drove inventors like Tesla and Edison to push the boundaries of existing technology.
The rivalry between Tesla’s AC and Edison’s DC, often referred to as the “War of Currents,” epitomised the era’s quest for a more efficient and practical means of power distribution. This competition spurred significant innovations in power generation, transmission, and conversion technologies, laying the foundation for the modern electrical grid.
The industrial boom of this period necessitated advancements in motor control and electrical machinery, further driving the development of power electronics. The societal shift towards electrification called for robust systems capable of converting and controlling power in a reliable and efficient manner, setting the stage for the technological leaps that would follow in the field of power electronics.
The Dawn in the History of Power Electronics
The inception of power electronics can be traced back to the challenges faced in the early days of electricity distribution. The foundational debate between alternating current (AC) and direct current (DC) systems played a pivotal role in shaping the future of electrical power systems.
Edison’s Direct Current (DC) Advocacy
Thomas Edison, a proponent of direct current, championed DC for its simplicity and the immediate control it offered over electrical power. Edison’s DC systems were the first to be deployed in urban settings, powering streetlights and homes with electricity generated from central power stations. However, DC’s major drawback was its inability to be efficiently transmitted over long distances. The power loss associated with DC transmission lines was substantial, necessitating the construction of power generation sites in close proximity to consumption areas, which was not always practical or economically feasible.
Tesla’s Alternating Current (AC) Vision
In contrast, Nikola Tesla saw the potential of alternating current to overcome the limitations of DC. Tesla, along with George Westinghouse, advocated for AC because of its ability to be easily transformed to higher or lower voltages. This characteristic of AC made it possible to transmit power over long distances with significantly reduced losses, a game-changer for the burgeoning demand for electrical power. Tesla’s development of the AC induction motor and the polyphase AC system further demonstrated the versatility and efficiency of AC, leading to its widespread adoption for power distribution.
The AC/DC Debate and Its Impact
The debate between AC and DC was more than a technical dispute; it was a battle that defined the path of electrical distribution systems. Edison’s initial success with DC systems in urban centres set the stage, but it was Tesla’s AC systems that ultimately revolutionised power distribution on a larger scale. The ability to transmit power over long distances without substantial loss opened up new possibilities for the electrification of society, from rural electrification to the development of large-scale power plants.
This early period in the history of power electronics highlighted the critical importance of efficient power conversion and control. The challenges and solutions of this era laid the groundwork for the field of power electronics, setting the stage for future innovations in power conversion, distribution, and management.
Legacy and Future Directions
While AC became the dominant standard for power distribution, the story didn’t end there. The advent of solid-state electronics and the development of advanced power conversion technologies have led to a resurgence of DC in many applications, from datacentres to renewable energy systems and electric vehicles. Modern power electronics now focus on the seamless conversion between AC and DC, leveraging the strengths of both to meet the demands of contemporary electrical systems.
The AC vs. DC battle, therefore, was not just a historical footnote but a foundational episode that continues to influence the field of power electronics, driving innovations in efficient power conversion, energy storage, and smart grid technologies.
The Mercury Arc Rectifier Era
The advent of the mercury arc rectifier marked a significant milestone in the history of power electronics, introducing a viable solution for converting alternating current (AC) to direct current (DC). This breakthrough technology emerged in the early 20th century, revolutionising power distribution and paving the way for the development of early power electronics.
Mercury Arc Rectifier is at Belsize Park’s deep-level shelter in London. Photo by C Ford. Secretlondon, CC BY-SA 3.0, via Wikimedia Commons
Breakthrough in AC to DC Conversion
The mercury arc rectifier utilised a pool of mercury and an arc discharge to convert AC to DC. When an electric arc was struck between a carbon anode and a mercury cathode, it ionised the mercury, creating a vapour that allows current to flow more easily in one direction (as the carbon anode cannot emit electrons well), thereby rectifying the AC input to DC output. This process was significantly more efficient than mechanical rectifiers or earlier electrochemical methods, offering a reliable and effective means of conversion for various applications.
Impact on Power Distribution
The introduction of the mercury arc rectifier had a profound impact on power distribution networks, particularly in industries and transportation systems that required substantial DC power. One of the most notable applications was in the electrification of railway systems. Many railway networks, especially in urban areas, operated on DC power, and the mercury arc rectifier enabled the efficient conversion of AC from the main power grid to the DC needed for traction systems.
Additionally, mercury arc rectifiers were instrumental in electrochemical processes, such as aluminium production, where large amounts of DC power were essential. Their ability to handle high power levels and voltages made them indispensable in industrial settings, contributing to the growth and efficiency of various manufacturing sectors.
Role in Early Power Electronics
The era of the mercury arc rectifier represents an early chapter in the field of power electronics, highlighting the ongoing quest for efficient power conversion technologies. While the basic principle of rectification was not new, the mercury arc rectifier’s efficiency and capacity for high-power applications demonstrated the potential of electronic solutions for power conversion challenges.
This technology also set the stage for further innovations in power electronics, as engineers and scientists sought to improve upon the mercury arc rectifier’s performance and overcome its limitations, such as the maintenance required for the mercury vapour environment and the eventual development of more compact and environmentally friendly solid-state devices.
The legacy of the mercury arc rectifier era is evident in the continuous evolution of power conversion technologies, from vacuum tubes and thyristors to modern semiconductor devices like diodes and transistors, which now form the backbone of contemporary power electronics systems.
Vacuum Tubes: Controlling Electricity Flow
The development of vacuum tubes in the early 20th century represented a pivotal advancement in the control and amplification of electrical signals, marking a significant era in the evolution of power electronics. These devices, consisting of sealed glass or metal tubes with electrodes inside a vacuum, became the first reliable method to control the flow of electricity in electronic circuits.
CK512AX compared to standard vacuum tubes. Mister rf, CC BY-SA 4.0, via Wikimedia Commons
Development and Significance
Vacuum tubes, or thermionic valves as they were also known, operated by controlling the flow of electrons from a heated cathode to an anode within the vacuum. This electron flow could be modulated by introducing a grid between the cathode and anode, allowing for the amplification and switching of electrical signals. The ability to control the flow of electricity in this manner was revolutionary, enabling the development of early electronic devices such as radios, televisions, and the first computers.
The significance of vacuum tubes extended beyond these applications; they were instrumental in the early stages of power electronics, where controlling and modulating power was crucial. Vacuum tubes allowed for the precise control of high-voltage electrical signals, making them suitable for applications in telecommunications, radar systems, and in the burgeoning field of electronic computing.
Paving the Way for Sophisticated Systems
Vacuum tubes laid the groundwork for more sophisticated power electronic systems by demonstrating that electronic devices could effectively control and manipulate electrical power. This realisation spurred further research and development in the field, leading to the exploration of new materials and technologies to improve the efficiency, reliability, and performance of electronic control systems.
The key limitations of vacuum tubes, however, was their size, fragility, and the heat they generated, which spurred the search for more durable, compact, and efficient alternatives. This quest ultimately led to the invention of the semiconductor transistor, a smaller, more reliable device that consumed less power and heralded a new era in electronics, including power electronics.
The transition from vacuum tubes to solid-state devices marked a significant evolution in the field, enabling the miniaturization and sophistication of electronic systems. The principles of controlling and amplifying electrical signals developed with vacuum tubes, however, remain foundational to the design and operation of modern power electronic systems, from consumer electronics to industrial control systems and beyond.
The legacy of vacuum tubes in power electronics is a testament to the importance of innovation in controlling electricity flow, a principle that continues to drive advancements in the field, leading to more efficient, reliable, and powerful electronic systems.
The Semiconductor Revolution
The transition from vacuum tubes to semiconductors marked a transformative era in power electronics, heralding unprecedented advancements in the efficiency, size, and functionality of electronic devices. This shift not only revolutionized the field of power electronics but also laid the foundation for the modern digital age.
Transition to Semiconductors
The discovery and development of semiconductor materials, such as silicon and germanium, enabled the creation of the transistor in the late 1940s. Unlike vacuum tubes, transistors were smaller, more reliable, and required less power to operate. This made them ideal for a wide range of applications, from consumer electronics to complex computing systems. The inherent efficiency and durability of semiconductors allowed for the miniaturization of circuits and the development of portable electronic devices, fundamentally changing the landscape of technology.
Early Challenges
Despite their potential, early semiconductor devices faced significant challenges. The initial production of semiconductor materials and devices was fraught with difficulties related to purity and consistency, which impacted the performance and reliability of the devices. Moreover, the understanding of semiconductor physics was still evolving, necessitating extensive research and development to optimise the design and fabrication of semiconductor devices.
The development of the integrated circuit (IC) in the late 1950s and early 1960s represented a major breakthrough, allowing multiple transistors and other components to be fabricated on a single silicon chip. This innovation dramatically increased the complexity and capability of electronic systems while reducing their cost and size.
Evolution of Performance and Applications
As the understanding of semiconductor materials and device physics advanced, so too did the performance and applications of semiconductor devices. The introduction of doping techniques to modulate the electrical properties of semiconductors, the development of metal-oxide-semiconductor field-effect transistors (MOSFETs), and the advent of complementary metal-oxide-semiconductor (CMOS) technology were pivotal in enhancing the efficiency and functionality of semiconductor devices.
These advancements expanded the scope of power electronics into areas previously unimagined, from the control and conversion of electrical power in industrial systems to the management of energy in renewable sources and electric vehicles. The scalability and versatility of semiconductor devices have made them indispensable in the design of modern power electronic systems, enabling precise control over a wide range of power levels and applications.
The semiconductor revolution transformed power electronics from a field concerned primarily with the basic control and conversion of electrical power into a dynamic and integral component of all modern electronic systems. This revolution continues today, with ongoing innovations in semiconductor technology driving further advancements in power electronics, including the development of wide-bandgap semiconductors like silicon carbide (SiC) and gallium nitride (GaN), which promise even greater efficiency and performance in power electronic systems.
Technological Challenges and Solutions in Power Electronics
The evolution of power electronics has been marked by a series of technological challenges, each pushing the boundaries of materials science, component design, and system integration. Addressing these challenges has been crucial for advancing the field and expanding the capabilities of power electronic systems.
Efficiency Losses
One of the perennial challenges in power electronics has been minimizing energy loss during power conversion and control processes. Losses typically manifest as heat, which not only reduces the overall efficiency of the system but also impacts reliability and lifespan. Early power electronic systems, reliant on bulkier components like vacuum tubes and mechanical switches, were particularly prone to significant efficiency losses.
Solutions: The transition to semiconductor-based devices, such as transistors and diodes, marked a significant leap in addressing efficiency concerns. Semiconductors offered much lower resistance paths and faster switching capabilities, drastically reducing energy losses. Further advancements in semiconductor technology, including the development of MOSFETs and later, wide-bandgap materials like SiC and GaN, have continued to push the efficiency envelope, enabling devices that can operate at higher frequencies, voltages, and temperatures with reduced losses.
Heat Management
As power electronic devices became capable of handling higher power densities, managing the resultant heat became increasingly challenging. Excessive heat can lead to device failure, reduced performance, and reliability issues, necessitating effective thermal management solutions.
Solutions: Innovations in heat dissipation techniques have been critical in addressing thermal management challenges. These include the development of advanced heat sinks, liquid cooling systems, and thermal interface materials designed to efficiently remove heat from power electronic devices. The integration of these thermal management solutions into device and system design has been essential for maintaining performance and reliability in high-power applications.
Material Limitations
The performance and capabilities of power electronic systems are inherently tied to the properties of the materials from which they are made. Traditional semiconductor materials like silicon, while revolutionary, have physical limitations, particularly at high voltages and temperatures.
Solutions: The exploration and adoption of wide-bandgap semiconductor materials, such as silicon carbide (SiC) and gallium nitride (GaN), have provided significant breakthroughs in overcoming the limitations of silicon. These materials offer superior electrical and thermal properties, allowing for devices that can operate at higher voltages, temperatures, and switching speeds, further enhancing efficiency and reducing size.
System Integration
As power electronic systems grew in complexity, integrating various components into cohesive, reliable systems presented significant challenges. This was particularly evident in the development of sophisticated applications like renewable energy systems, electric vehicles, and smart grids, where the seamless integration of power generation, conversion, storage, and control components is critical.
Solutions: Advances in digital control technologies and the development of modular design approaches have greatly facilitated system integration. Digital signal processors (DSPs) and microcontrollers now enable precise control over power electronic systems, allowing for adaptive and intelligent management of power flows. Modular designs, where components are designed as interchangeable units, have simplified system assembly and maintenance, enhancing flexibility and scalability.
The journey of power electronics from its early days to the present has been a continuous cycle of identifying challenges and innovating solutions. Each challenge addressed has paved the way for new applications and capabilities, driving the relentless advancement of the field. As power electronics continues to evolve, the focus on efficiency, reliability, and integration remains paramount, with ongoing research and development aimed at overcoming the next set of challenges.
Miniaturization and Efficiency in Power Electronics
The relentless drive towards miniaturization and efficiency in power electronics has been significantly influenced by advancements in semiconductor technology. The ability to shrink the size of power electronic components without compromising their performance has revolutionized the field, enabling a new era of compact, efficient devices across a wide range of applications.
Sophisticated High-Precision Robotic Arm in a Well-Lit Electronics Manufacturing Facility. Industry for Producing Electronic Devices. Automated Installation of Components on Circuit Boards. State-of-the-Art, Fully Automated PCB Assembly Line.
Role of Semiconductors in Miniaturization
Semiconductors have been at the forefront of the miniaturization trend in power electronics. The transition from bulky, inefficient components like vacuum tubes to compact, efficient semiconductor devices marked the beginning of this transformation. Semiconductors, with their small size and low power requirements, allowed for the development of much smaller and more efficient power electronic systems.
The invention of the integrated circuit (IC) was a pivotal moment in this journey, allowing multiple semiconductor devices to be fabricated on a single silicon chip. This not only reduced the physical size of electronic circuits but also significantly improved their reliability and performance by minimizing the connections between components.
Advancements in Device Efficiency
The efficiency of power electronic devices has seen remarkable improvements alongside miniaturization efforts. Early semiconductor devices, while more efficient than their predecessors, still faced limitations in terms of power handling and efficiency losses. The development of advanced semiconductor materials and fabrication techniques has been key to overcoming these challenges.
Wide-bandgap semiconductors like silicon carbide (SiC) and gallium nitride (GaN) have emerged as game-changers in this regard. Their superior electrical and thermal properties allow for devices that can operate at higher voltages and temperatures with greater efficiency, further reducing size and cooling requirements.
Impact on Device Functionality
The miniaturization and enhanced efficiency of power electronic components have had a profound impact on device functionality. Smaller, more efficient components have enabled the integration of power electronics into a vast array of devices, from portable consumer electronics to electric vehicles and renewable energy systems.
In consumer electronics, the miniaturization of power electronic components has been instrumental in the development of compact, portable devices with extended battery life and improved performance. In the automotive sector, advanced power electronics have been key to the development of electric and hybrid vehicles, where efficient, compact power conversion and management systems are critical for performance and range.
In renewable energy systems, miniaturized, efficient power electronic components have enabled the development of more effective energy conversion and storage solutions, facilitating the integration of renewable sources into the power grid.
Future Directions
The trends of miniaturization and efficiency in power electronics continue to drive innovation in the field. Ongoing research is focused on further advancements in materials science, component design, and system integration to develop even smaller, more efficient power electronic systems. These efforts aim to address the growing demand for energy-efficient, high-performance electronic devices and systems, paving the way for new applications and technologies in an increasingly electrified world.
The role of miniaturization and efficiency in the evolution of power electronics underscores the field’s dynamic nature, where continuous advancements in technology lead to ever more compact, efficient, and capable power electronic systems.
The MOSFET Breakthrough
Two MOSFETs in D2PAK package, each rated at 30-A, 120-V. CyrilB~commonswiki assumed (based on copyright claims)., CC BY-SA 3.0, via Wikimedia Commons
The Metal-Oxide-Semiconductor Field-Effect Transistor (MOSFET) represents one of the most significant breakthroughs in the field of power electronics. Since its introduction, the MOSFET has revolutionized the design and functionality of power electronic systems, offering unprecedented levels of isolation, efficiency, and control.
Introduction to MOSFET
The MOSFET is a type of field-effect transistor (FET) that controls the flow of current using an electric field to modulate the conductivity of a channel. Its structure consists of a source, drain, and gate, where the gate terminal is electrically insulated from the main current-carrying channel by a thin oxide layer. This insulation provides the MOSFET with high input impedance and low power consumption, distinguishing it from other transistor types.
Revolutionary Impact on Power Electronics
Improved Isolation: The insulated gate of the MOSFET provides excellent electrical isolation between the control circuit (gate) and the power circuit (source-drain). This feature significantly reduces the risk of feedback from the power circuit affecting the control circuit, enhancing the reliability and stability of power electronic systems, while also offering a degree of electrical isolation..
Enhanced Efficiency: MOSFETs are known for their high switching speed and low on-resistance, which contribute to reduced power loss during operation. The ability to switch rapidly without significant energy dissipation makes MOSFETs ideal for applications requiring efficient power conversion and management, such as in switch-mode power supplies (SMPS), inverters, and DC-DC converters.
Precise Control: The high input impedance and fast switching capabilities of MOSFETs allow for precise control over the flow of current in power electronic circuits. This precise control is crucial in applications where fine adjustments to power output are necessary, such as in motor drives and power regulators.
Applications and Advancements
The versatility and superior performance of MOSFETs have led to their widespread adoption across a broad spectrum of power electronic applications. In the realm of renewable energy, MOSFETs are integral to the efficient conversion of energy in solar inverters and wind turbine controllers. In the automotive industry, they play a critical role in the power management systems of electric and hybrid vehicles, contributing to improved energy efficiency and vehicle performance.
Over time, advancements in MOSFET technology have further enhanced their performance and applicability. The development of power MOSFETs, designed to handle higher currents and voltages, has expanded their use in high-power applications. Innovations such as superjunction MOSFETs have addressed the limitations of conventional MOSFET designs, offering even lower on-resistance and higher efficiency.
Future Perspectives
The ongoing evolution of MOSFET technology continues to push the boundaries of power electronics, with research focused on improving the material properties, device structures, and fabrication techniques. The integration of wide-bandgap materials like SiC and GaN in MOSFET design is particularly promising, offering the potential for devices that can operate at even higher efficiencies, frequencies, and temperatures.
The MOSFET breakthrough has fundamentally shaped the landscape of power electronics, providing a robust and versatile component that has enabled a multitude of technological advancements. As the field of power electronics progresses, the MOSFET’s role as a cornerstone technology remains undisputed, with its continued evolution central to the development of more efficient, reliable, and high-performance power electronic systems.
The Advent of IGBTs
The development of Insulated Gate Bipolar Transistors (IGBTs) represents a significant milestone in the field of power electronics, merging the advantages of Metal-Oxide-Semiconductor Field-Effect Transistors (MOSFETs) and Bipolar Junction Transistors (BJTs) to handle higher power applications with greater efficiency.
Combining MOSFETs with BJTs
IGBTs combine the gate-drive characteristics of MOSFETs with the high-current and low-saturation-voltage capabilities of BJTs. This hybrid structure allows IGBTs to offer the ease of control of MOSFETs while being able to sustain high voltage and current levels typical of BJTs. The insulated gate of the IGBT provides excellent input impedance and fast switching speeds, while the bipolar nature of the device ensures robust performance under high-power conditions.
Significance in Power Electronics
Greater Power Handling: IGBTs are designed to handle very high voltages and currents, making them particularly suited for high-power applications such as industrial motor drives, electric vehicle powertrains, and power grid systems. Their ability to efficiently switch and amplify large power levels has made them a cornerstone in the design of modern power electronic systems.
Enhanced Efficiency: The efficiency of power electronic systems is significantly improved with the use of IGBTs, thanks to their low on-state voltage drop and fast switching capabilities. These characteristics minimize power losses during operation, leading to more efficient power conversion processes, especially in applications requiring high voltage and current handling capabilities.
Improved Thermal Performance: IGBTs exhibit better thermal stability compared to their MOSFET counterparts, especially in high-power applications. This improved thermal performance is crucial in reducing the need for extensive cooling systems, thereby simplifying system design and reducing overall costs.
Applications and Advancements
IGBTs have found widespread use in a variety of power electronic applications, from renewable energy systems and electric vehicles to high-speed trains and power grid stabilizers. Their robustness and efficiency have enabled the development of more reliable and higher-performing systems across these sectors.
Ongoing advancements in IGBT technology continue to enhance their performance and applicability. Developments in semiconductor materials, device architectures, and packaging technologies have led to IGBTs with lower losses, higher switching frequencies, and improved reliability. The integration of wide-bandgap materials like silicon carbide (SiC) into IGBT structures is also being explored to further push the boundaries of their performance.
Future Perspectives
The advent of IGBTs has had a transformative impact on power electronics, enabling the efficient management and conversion of high levels of power. As the demand for energy-efficient and high-power electronic systems continues to grow, the role of IGBTs in meeting these demands is increasingly significant. The ongoing evolution of IGBT technology, driven by advancements in materials science and semiconductor engineering, promises to further expand the capabilities of power electronic systems, paving the way for more sustainable and efficient energy solutions.
Electrification and Power Electronics
The global push towards electrification, driven by the urgent need to transition away from non-renewable energy sources and mitigate climate change, has underscored the increasing importance of power electronics. As societies move towards more sustainable energy solutions, the role of power electronics in enabling and optimizing these technologies has become paramount.
Facilitating the Shift to Renewable Energy
Power electronics are at the heart of renewable energy systems, including solar photovoltaic (PV) panels, wind turbines, and hydroelectric plants. These systems rely on power electronic converters to efficiently convert and manage the electricity they generate. For instance, solar inverters convert the DC power produced by solar panels into AC power that can be fed into the electrical grid or used by AC-powered devices. Similarly, power electronics in wind turbines convert and regulate the variable frequency and amplitude of the electricity generated by the turbine into a stable form that is compatible with the grid.
Enhancing Energy Efficiency
Advancements in power electronics not only facilitate the integration of renewable energy sources into the grid but also enhance the overall efficiency of electrical systems. High-efficiency power converters minimize energy losses during the conversion process, ensuring that a greater proportion of the generated renewable energy is utilized. This efficiency is crucial in making renewable energy sources more competitive with traditional fossil fuels and in reducing the overall energy consumption of electrical systems.
Enabling Electrification of Transportation
The electrification of transportation, a key component of the global electrification movement, is heavily reliant on power electronics. Electric vehicles (EVs), from passenger cars to buses and trains, depend on power electronic systems for various functions, including battery management, motor control, and energy regeneration. The efficiency, reliability, and performance of EVs are directly influenced by the advancements in power electronics, making them a critical factor in the widespread adoption of electric transportation.
Supporting Smart Grids and Energy Storage
The transition to a more electrified world also involves the development of smart grids and advanced energy storage solutions, both of which are enabled by power electronics. Smart grids use power electronic converters to manage and direct the flow of electricity in a more efficient, reliable, and flexible manner, accommodating the variable nature of renewable energy sources. Power electronics also play a crucial role in energy storage systems, converting and regulating the power stored in batteries or other storage media for use when demand is high or generation is low.
Future Outlook
As the global push towards electrification gains momentum, the importance of power electronics continues to grow. Ongoing research and development in this field are focused on creating more efficient, compact, and robust power electronic devices and systems that can meet the challenges of a rapidly electrifying world. Innovations in semiconductor materials, device architectures, and system integration are key to achieving these goals, ensuring that power electronics remain at the forefront of the electrification movement and the transition towards a more sustainable and energy-efficient future.
The Future: GaN and SiC Technologies
The future of power electronics is being shaped by the emergence of Gallium Nitride (GaN) and Silicon Carbide (SiC) technologies. These wide-bandgap semiconductors are set to redefine the landscape of power systems with their superior performance characteristics, offering significant advantages over traditional silicon-based technologies.
MicroLED arrays processed with GaN-on-Silicon technology on a wafer. Palee93, CC BY-SA 4.0, via Wikimedia Commons
Introduction to GaN and SiC
Gallium Nitride (GaN): GaN is a wide-bandgap semiconductor material known for its high electron mobility, thermal conductivity, and ability to operate at higher temperatures and voltages than silicon. These properties make GaN devices particularly suitable for high-frequency, high-efficiency applications. GaN technology is increasingly being used in power electronic systems such as power adapters, chargers, and RF power amplifiers, where its efficiency and size advantages are most pronounced.
Silicon Carbide (SiC): SiC is another wide-bandgap material that has been gaining attention in the power electronics community. It is known for its high thermal conductivity, high electric field breakdown strength, and high maximum current density. SiC devices, such as diodes, MOSFETs, and IGBTs, are well-suited for high-power applications like electric vehicles, industrial motor drives, and renewable energy systems, where they can significantly improve efficiency, thermal performance, and system reliability.
Potential Impacts on Power Systems
The adoption of GaN and SiC technologies has the potential to revolutionize traditional power systems in several ways:
Increased Efficiency: Both GaN and SiC devices exhibit lower conduction and switching losses compared to their silicon counterparts, leading to higher overall system efficiencies. This efficiency gain is crucial in applications where energy conservation and thermal management are critical, such as in renewable energy systems and electric vehicles.
Higher Power Density: The superior thermal and electrical properties of GaN and SiC allow for the design of power electronic components that are smaller and lighter than those made from silicon, without compromising performance. This increase in power density is particularly beneficial in portable and space-constrained applications.
Enhanced Thermal Performance: The high thermal conductivity of SiC and the high-temperature tolerance of GaN enable these devices to operate effectively at higher temperatures. This reduces the need for extensive cooling systems, simplifying design and reducing costs.
Improved Reliability: GaN and SiC devices demonstrate enhanced reliability, especially in harsh environments, due to their robust physical and chemical properties. This reliability is essential in critical applications such as aerospace, defense, and automotive systems.
Future Outlook
As GaN and SiC technologies continue to mature, their adoption is expected to accelerate, further displacing traditional silicon-based devices in a wide range of power electronic applications. Ongoing research and development efforts are focused on overcoming the current challenges associated with these materials, such as manufacturing costs and device packaging, to fully unlock their potential.
The future of power electronics, driven by GaN and SiC technologies, promises not only more efficient and compact power systems but also a significant step forward in the global transition towards cleaner, more sustainable energy solutions. The potential impacts of these advanced semiconductor technologies are vast, heralding a new era of performance and innovation in power electronic systems.
Conclusion
The journey detailed in this history of power electronics, from its nascent stages to today’s cutting-edge technologies, is a testament to relentless innovation and efficiency in the field of electrical engineering. This journey has been marked by several key milestones, each representing a leap forward in our ability to control, convert, and manage electrical power with greater precision and efficiency.
Recap of Milestones
-
The AC vs. DC Era: The foundational debate between alternating current (AC) and direct current (DC) systems set the stage for the development of power electronics, highlighting the need for efficient power conversion and distribution methods.
-
The Advent of Semiconductor Devices: The transition from mechanical switches and vacuum tubes to semiconductor devices like diodes, transistors, and eventually integrated circuits (ICs) revolutionized power electronics, enabling miniaturization and enhanced performance.
-
The MOSFET Breakthrough: The development of the Metal-Oxide-Semiconductor Field-Effect Transistor (MOSFET) brought unprecedented levels of control and efficiency to power electronic systems, paving the way for their widespread adoption in a variety of applications.
-
The Introduction of IGBTs: Insulated Gate Bipolar Transistors (IGBTs) combined the best attributes of MOSFETs and bipolar junction transistors (BJTs), offering a solution for high-power applications that require both high efficiency and robust performance.
-
The Rise of Wide-Bandgap Semiconductors: The emergence of Gallium Nitride (GaN) and Silicon Carbide (SiC) technologies has ushered in a new era of power electronics, characterized by even higher efficiencies, greater power densities, and improved thermal performance.
Ongoing Evolution and Future Prospects
The evolution of power electronics is far from over. As the world continues to grapple with the challenges of climate change and the need for sustainable energy solutions, the role of power electronics becomes increasingly critical. The ongoing advancements in semiconductor technologies, component design, and system integration are driving the development of more efficient, reliable, and compact power electronic systems that are essential for harnessing renewable energy, electrifying transportation, and improving energy efficiency across a broad spectrum of applications.
The future prospects of power electronics lie in the continued innovation in materials science, the exploration of new device architectures, and the integration of power electronic systems with digital technologies to create intelligent, adaptive solutions. These advancements hold the promise of not only driving technological innovation but also playing a pivotal role in the global transition towards a more sustainable and electrified future.
In conclusion, the field of power electronics stands at the forefront of technological progress, with its ongoing evolution poised to make significant contributions to both our technological capabilities and our sustainability goals. The journey of power electronics, from its humble beginnings to the forefront of high-tech innovation, reflects the ingenuity and perseverance of engineers and scientists dedicated to improving the way we generate, use, and manage electrical power.
References and Further Reading
For those interested in delving deeper into the rich history and technical intricacies of power electronics, the following list of authoritative sources offers a comprehensive starting point. These references encompass seminal texts, scholarly articles, and industry standards that have shaped the understanding and advancement of power electronics.
“Power Electronics: Converters, Applications, and Design” by Ned Mohan, Tore M. Undeland, and William P. Robbins.
-
A foundational textbook offering a comprehensive overview of power electronic converters, applications, and design considerations.
“Principles of Power Electronics” by John G. Kassakian, Martin F. Schlecht, and George C. Verghese.
-
This book provides an in-depth treatment of the principles and techniques of modern power electronics, suitable for advanced undergraduate and graduate students.
“Semiconductor Power Electronics” by Robert W. Erickson.
-
Focused on the semiconductor devices used in power electronics, this book covers the principles, design, and applications of these critical components.
IEEE Transactions on Power Electronics.
-
A leading journal offering the latest research findings in power electronics, including advancements in semiconductor technologies, converter topologies, and applications.
“Wide Bandgap Power Semiconductor Packaging: Materials, Components, and Reliability” by Katsuaki Suganuma.
-
This book explores the packaging technologies for wide-bandgap semiconductors, addressing materials, components, and reliability aspects critical for GaN and SiC devices.
“High-Power Converters and AC Drives” by Bin Wu.
-
A detailed resource on high-power converters and AC drive systems, highlighting the role of IGBTs and other power electronic devices in industrial applications.
“Gallium Nitride (GaN): Physics, Devices, and Technology” by Farid Medjdoub.
-
Offering a comprehensive overview of GaN technology, this book covers the physical properties, device fabrication, and applications of GaN in power electronics.
“Silicon Carbide Power Devices” by B. Jayant Baliga.
-
This book delves into SiC power devices, covering their physical properties, fabrication techniques, and applications in power electronic systems.
“The Power Electronics Handbook” edited by Timothy L. Skvarenina.
-
A broad reference covering the theory, design, and applications of power electronic systems, suitable for engineers and professionals in the field.
“Advanced Power Electronics Converters: PWM Converters Processing AC Voltages” by Euzeli dos Santos Jr. and Edison R. da Silva.
-
This text focuses on advanced PWM converters for processing AC voltages, offering insights into the design and operation of these critical components in power systems.